Contents
2. Navigation Principles
2.1 Static Guidance
2.2 Dynamic Guidance
3. Intraoperative MR Systems for Functional Imaging
4. References
1.
Introduction
Due to the
high soft tissue contrast, the absence of ionizing radiation and
spatially unrestricted
imaging capabilities, MRI has become the
predominant imaging modality for neurosurgical interventions. MRI-based
navigation techniques assist the neurosurgeon with the orientation at
the
patient and allow precise instrument guidance.
Navigation in neurosurgery can be roughly divided into preoperative and intraoperative based approaches. In preoperative based approaches, MRI images are acquired several days prior to surgery and co-registered (i.e., mapped to the spatial coordinates of the patient during surgery in relation to the intervention position). Even though these methods are not restricted in terms of the magnetic field and offer the entire diagnostic capabilities of MR, they do not allow for adjustment of brain shift, that occurs when the cranium is opened. This technical inability has led to intraoperative MR systems, that acquire MRI images during surgery. The specific advantages and limitations of these systems are highly dependent on the magnet design that defines the diagnostic applications and the interventional environment.
2. Navigation Principles
Navigation generally means the use of geometrical an anatomical data to approach a target position in or at the patient. For this purpose the position and orientation of a surgical instrument needs to be reliably defined. A stereoscopic camera is typically used to continuously determine the 3D position of an instrument.
Several navigation techniques and the corresponding MR-systems are briefly discussed bellow:
2.1 Static Guidance
Frame based stereotaxy [1] without intraoperative control is the simplest form of navigation and is performed in a traditional operating room based on preoperative MRI data. It is most commonly used for interventions, where brain shift is not critical, or for biopsy procedures.
An MR-compatible frame with MR-visible markers is securely fixed to the skull prior to MR imaging. After scanning, the corresponding data are transferred to the OR, where the surgical procedure is planned. In addition, the MR-visible markers need to be identified on the MR image. After that, the corresponding coordinates at the frame are computed and the guidance system is set accordingly. The surgical intervention may then be performed in the predetermined manner as long as no intraoperative changes occur.
The aim
of dynamic guidance
is to control the slice orientation in such a way that the instrument
is
ideally displayed along its entire length, (i.e., on an oblique slice
defined
by the actual orientation of the instrument).
One
common and
relatively simple approach is called freehand navigation. However, that
term is
differently defined for interventions in either closed-bore or open MR
scanners.
In closed-bore systems the instrument is iteratively advanced to the
target by
switching between manipulation outside the bore and control imaging
inside the
bore. In open MR scanners a suitable plane including the target point
is
defined and continuously scanned. If the orientation of the instrument
is
tracked, then the selected plane may be adapted automatically.
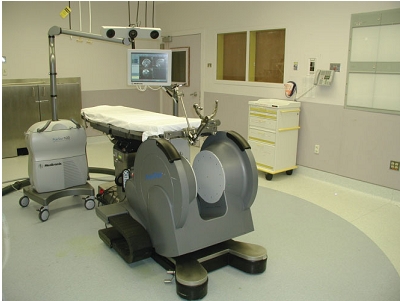
Fig 1. A 0.12 T intraoperative MR system, where surgery is performed entirely within the magnetic field using standard instrumentation [2]. |
Another system for navigation, based on continuous imaging, is shown in Fig.2. This vertically open 0.5 T system, does not necessariyily require coregistration between patient and image data, because the stereoscopic camera can be integrated within the MR scanner.
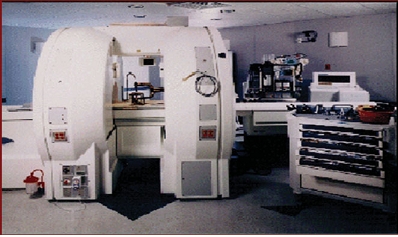
Fig. 2. A 0.5 T double
coil design intraoperative MR system, where imaging can be performed continuously
while the surgeon operates between the coils [2]. |
3. Intraoperative MR Systems for Functional Imaging
Many of the MR techniques for functional neuroimaging, including MR venography (MRV), MR angiography (MRA), brain activity studies known as functional magnetic resonance imaging (fMRI), and diffusion-weighted imaging (DWI), require magnetic field strength of at least 1.5 T. High field strengths are especially necessary for applications where quantitative accuracy is important and where signal to noise ratio (SNR) is critical. Since SNR is the limiting factor for DTI measures, a high magnetic field stenght of at least 1.5 T is required for adequate DTI measuremts. At this point, high field strengths of 1.5 T and above, only can be achieved with closed-bore MR systems. Such a intraoperative closed-bore MR systems is shown in Fig. 3.
Fig. 3. A intraoperative 1.5 T closed-bore MR system, that can be used for functional imaging during neurosurgery [2]. |