Principles of Single-Cell Analysis
Drug discovery and implementation depend heavily on how cells respond to a certain stimulus. Unfortunately, cell behavior differs from manmade input-output systems in that most cellular signaling pathways are dominated by stochastic processes. The obvious solution, then, is to analyze them on a cell-by-cell basis, generating a probability distribution of possible responses to a certain perturbation. However, we do not have any ideal means of simultaneously analyzing spatial- and temoral-dependent phenomenon, especially if we are aiming for speed and efficiency. Results are further complicated by varied environments: it proved exceedingly difficult to maintain a steady environment for every single cell during an experiment, thus introducing a major confounding variable. This complication was particularly severe in devices that attempted to replicate dynamic environments. Fortunately, the microfluidics community is developing means of overcoming all of the above challenges. Below is a brief summary of established techniques for single-cell assaying, as well as an introduction to the role that microfluidics might play in the near future.
Traditional techniques for cell studies
- Bulk methods:
This encompasses methods that maintain fairly constant environments across cells, but introduce a major complication by averaging parameter values, such as concentration of a certain marker or amount of fluorescence, across all data points, ignoring the possibility that some cells might be expressing nothing at all.
For example, as depicted at the right, if cells are switching from green to blue, at any given time, individual cells are either close to one color or the other, but the bulk assay mashes them into one average color and smoothes the individual transition curves into something not indicative of actual events.
|
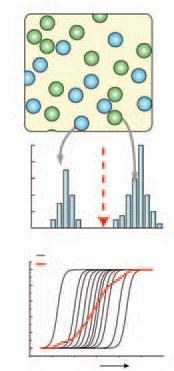
|
- Older single-cell methods:
- Flow Cytometry:
FC is a high-throughput technique whereby hydrodynamically-focused lines of cells zip through a laser that illuminates study-specific fluorescent markers placed in the cells. FC cannot map time-dependent phenomenon, and it is difficult to maintain a constant environment for the stream of cells. |
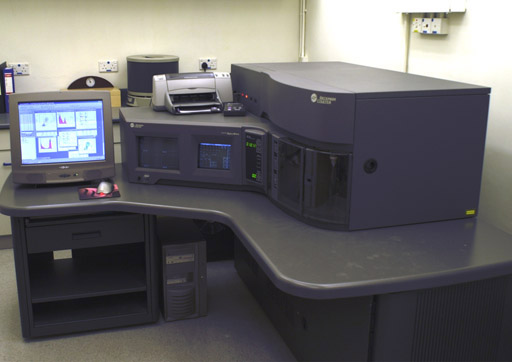 |
- Laser Scanning Cytometry:
This is similar to FC except that we move the laser instead of the cells. Because the cells are stationary, we can shine a laser on one cell only and observe any time-dependent processes. Unfortunately, it is a very time-consuming process to move the laser throughout the sample. |
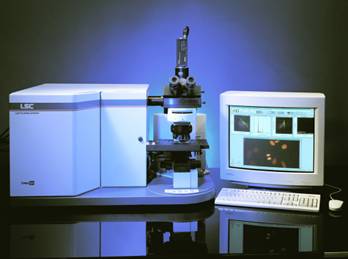 |
- Automated Microscopy:
This is a brute force method that uses a computerized microscope to take a massive series of photos of one fluorescently-labeled cell over time, which is then compiled by a computer. This allows us to carefully study cells in highly-controlled environment. It is an ungainly process though, and thus of little use to a clinician. |
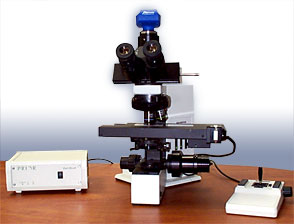 |
Non-Traditional Methods: The Near-Future
Borrowing a page from the world of 96-well microtiter plates and cDNA arrays, engineers began to develop high-throughput means of running single-cell assays while overcoming the challenges posed by existing methods. The surge in microfluidic technology due to names such as Whitesides and Quake has meant that over the last few years, fabrication and use of such devices have become simple enough that we can envision the day when researchers can fabricate them on-site and clinicians can purchase them cheaply. Such devices come in varying degrees of complexity, but whatever the intricacy, the general advantages remain constant.
Advantages of microfluidic cell assays over traditional means:
- High sensitivity
- Uniform microenvironments
- Efficient control of reagents
- Low cost of production
- High-content / high-throughput
Because of the above, there is currently a strong move towards the development of lab-on-a-chip technology for clinical use.
|
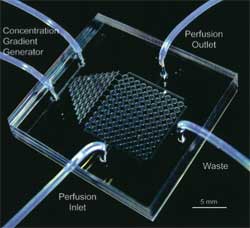 |
|