Current Applications
Below are a few of the current applications of bioprinting.
High throughput cell patterning
E.A. Roth et al. demonstrated the use of an automated printing process for the development of cellular patterns at a resolution in the range of 300-400µm. Simply modifying a commercial inkjet printer interfaced with a computer and basic software enables high-throughput capability and repeatability. Thermally driven, solution deposition onto the substrate occurs after the bioink heats to a threshold temperature range (between 200-300°C) forming a small bubble that forces a small droplet, of a controlled volume, out. The deposition of biomaterials such as collagen, the most common protein in the body, onto a cell repellent surface in combination with high-throughput inkjet technology enables precise cellular attachment and controlled proliferation resulting in desired and controlled cellular attachment patterns of varying complexity and multiple cell types. Some of the advantages of this approach include high-throughput capabilities, flexibility, use of nontoxic materials, low cost and minimal training required. This technology may enable more understanding of cellular interactions and tissue development and may serve as a vital stepping point towards the development of 3D structures.
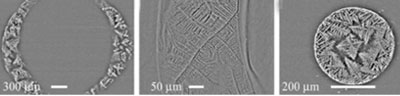
Images of example printed collagen patterns. Left two images are ring pattern at 40x and 100x magnification and right image displays circular pattern at 40x magnification.
Simultaneous printing for 3D tissue construction
One of the main proposals of bioprinting is the simultaneous printing of cells and biomaterials. Boland et al. demonstrated that this process allows for precise placement of cells and proteins onto 3D hydrogel structures (biopaper) which may further enable researchers to control spatial distribution, thickness of the 3D construct and differentiation of cells to desired tissue types. Applying a rapid gelling hydrogel system, many confluent layers of uniformly and homogeneously distributed cells and hydrogels can be printed into 3D tissue constructs. Cell patterning may enable the printing of pores or channels, of controlled uniform size, into the material to allow for the penetration of capillares and vessels.
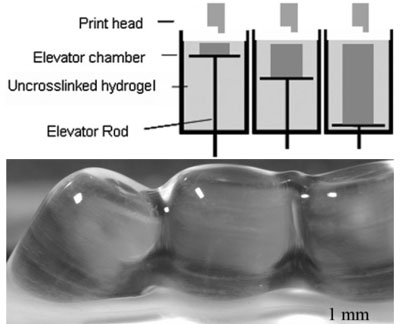
Schematic image of chamber of cell printer (top) and an example of printed structures (bottom).
The group developed a bioprinter with a moving platform inside the chamber as seen in the top image above. After each layer is printed, the elevator rod lowers resulting in the printed hydrogel structure on the stage when the rod reaches the bottom of the chamber. The bottom image shows printed parallel tubes immediately after printing (note that the stage is raised above liquid level).
TOP
Stem cell research and regenerative medicine
Phillippi et al. utilized bioprinting technology to engineer stem cell microenvironments. Using inkjet bioprinters, researchers were able to precisely control the placement of cells and thereby create spatially defined patterns of immobilized growth factors (bioinks) such as bone morphogenetic proteins (BMPs). By controlling placement and cell attachment patterns, the group attempted to direct stem cell fate, influencing the (mouse-derived) adult stem cells to differentiate into osteogenic or myogenic lineages. Their results demonstrated their ability to precisely control the spatial differentiation in direct correlation to the inkjet-printed patterns. Not only does this study lead to a better understanding of solid-phase patterns during different stages of tissue growth and remodeling (development, repair and regeneration), but may have potential clinical applications for regenerative medicine.
TOP
Tissue engineering patient-specific bone grafts
Following the three main steps in bioprinting, Hutmacher et al. demonstrates the potential of using bioprinting technologies for engineering bone grafts. In step 1, using medical imaging technology and computational modeling, CT scans capture the patient's bone defect and are used as data input to generate a computational 3D model. This computer-based model is then imported to a rapid prototyping system that divides the 3D rendered model into thin 2D horizontal slices. The information provided by this now sliced model provides the bioprinting device with layer-by-layer deposition instructions to construct a scaffold (i.e. shape and other anatomical features).
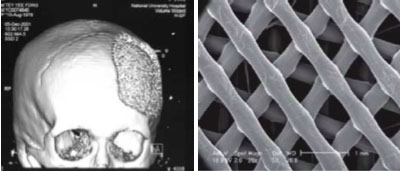
CT scan data of the patient's skull displaying the bone defect that was used to generate a blueprint or 3D model. The image on the right is the resulting scaffold or 3D tissue construct generated by the controlled layer-by-layer deposition using a bioprinter following bioprint.
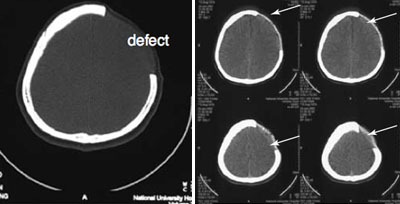
The images above demonstrate the usefulness and process of bioprinting biografts. The bottom-left image shows an example of a typical bone defect. The image on the right provides several examples of the application of this method. Although this may be better depicted in 3D rendered images, the custom-made scaffold and cell constructs, shown with the arrows, formed patient-specific bone grafts that accurately follow the complex 3D contour of the skull (bottom right).
TOP
Roadmap | Current Applications | Future Applications