Strategy #3: Stacking of Cell Monolayers (no scaffold material)
Another alternative strategy is to design cardiac muscle constructs without scaffold materials by stacking detached cardiac myocyte monolayers (called cell sheet engineering). This technique has a distinct advantage to the other strategies: different cell types could be stacked to yield a tissue construct of all cell species that make up the myocardium physiologically. This construct has no material-derived problems but it may not be as mechanically stable.
Research achievement with this strategy:
Shimizu , et al. Long-Term Survival and Growth of Pulsatile Myocardial Tissue Grafts Engineered by the Layering of Cardiomyocyte Sheets. Tissue Engineering 2006; 12(3):499-507.
The following image shows a layered patch of cardiomyocyte sheets. 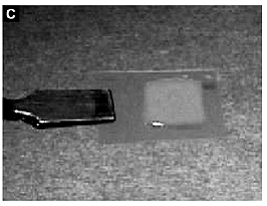
Shimizu and et al. have created a technique to create a three-dimensional spontaneously contractile myocardial tissue using cell sheet engineering by stacking layers of confluent cell sheets. The cells used are in this construct are neonatal rat ventricular myocytes. The cells are grown on temperature-sensitive polymer poly(N-isopropylacrylamide) (PIPAAm). This polymer is slightly hydrophobic and cell adhesive under normal culture conditions. However, when the temperature is reduced, the polymer becomes hydrophilic and releases the cells that were adhered to it. Thus, this type of surface can allow for cultured cells to be harvested easily without using digestive enzymes. This harvesting also allows for the extracellular matrix to be preserved and facilitates attachment to other cell sheets or tissues. After layered cardiomyocyte constructs are designed, they are transplanted into the dorsal subcutaneous tissues of nude rates and examined physiologically and morphologically for one year. After three days, microvascular networks were organizing within the grafts with sufficient neovascularization for blood supply and after seven days a well-organized vascular system was observed. The networks of microvasculation were preserved for up to one year.
In the following figure, these images show cross-sectional and macroscopic views of transplanted grafts at various time periods. A) Tissue observed in day 1 with red blood cell islands oriented randomly, B) At 3 days, the microvascular structures can be seen. C) At 7 days, several blood vessels are found. D) Macroscopic view at Day 3. E) At 7 days, a well-organized vascular system. F) Staining shows that the vascular system runs throughout the construct.
Size, conduction velocity, and contractile force of these constructs increased based on host growth, shown in following figure. Over time as the BW (body weight) increased in size, the other various properties of the constructs increased as well. Thus, these constructs have survived long term in vivo and have adapted to its environment.
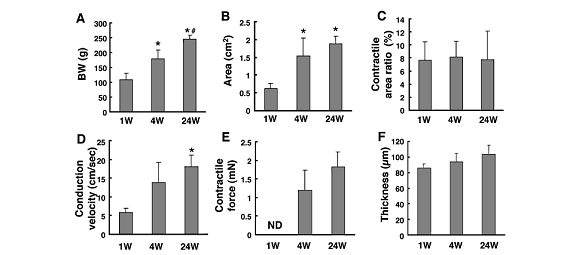
Histological analyses (following figure) illustrate that early formation of neovascular networks and well-differentiated cardiomyocytes with sarcomeres and gap junctions. Figure: A, D, G are at 1 week; B, E, H are at 4 weeks; C, F, I are at 24 weeks. The first row shows Azan staining of striated constructs and vessel and thickness growths. The middle row shows actinin staining of elongated cardiomyocytes and sarcomeres. The last row shows connexin 43 (gap junctions).
The contractile forces (4 mN) of in vivo layered cell sheets are smaller than those of whole hearts. Thus, this contractile force is likely to be insufficient in replacing heart function. However, this construct may be useful for myocardial tissue repair while further research is needed on vascularization of thicker tissues. |