Strategy #1: Seeding cells on synthetic or biological matrices
This strategy of seeding cells on synthetic or biological matrices is a classical approach in tissue engineering. The approach is to isolate specific cells and grow them on a three-dimensional scaffold under controlled culture conditions. Then, implant the construct in the body and direct new tissue formation into the scaffold. The scaffold temporarily replaces the extracellular matrix for the seeded cells until they can produce their own. The scaffold should be nontoxic, biodegradable and biocompatible. It also needs to match the biomechanical characteristics of the tissue it is replacing, porous, have adequate vascularization capabilities, and have the ability to release growth factors, gene signals, and/or other bioactive molecules. Three-dimensional scaffolds can serve to replace damaged tissue, provide a support for regeneration of tissues, and serve as a structure to guide the formation of tissues. Thus, the scaffold needs to provide physical support as well as chemical and biological cues to for organizing, growing and differentiating cells.
Materials used as scaffolds (Zammaretti P and Jaconi M. Cardiac Tissue Engineering: Regeneration of the Wounded Heart. Current Opinion in Biotechnology 2004; 15:430-434.)
Natural
Gelatin scaffolds |
Acellular porcine scaffolds |
Porous alginate scaffolds |
Alginate-gelatin-PEG scaffolds |
Collagen scaffolds |
Fibrin glue |
Advantages of natural polymers:
derived from the extracellular matrix; physiological components |
contain particular adhesive sequences on their surfaces that facilitate cell adhesion and cell differentiation. |
Disadvantages of natural polymers:
lack sufficient mechanical strength |
unless cross-linked chemically, they degrade rapidly in vivo |
inconsistent production |
potential contamination of materials from animal tissue |
Synthetic
PLA-PGA |
Poly-L-lactide-gelatin-PGA |
Electrically conducting membrane layers composed of PGA, gelatin, alginate and/or collagen |
Polyvinyl alcohol |
PGA-co-polyhydrobutyrates |
e-Caprolactone-co-L-lactide |
polyurethanes |
TMC-co- e-caprolactone-co-D-L-lactide |
Advantages of synthetic polymers:
High level of control over chemical and mechanical properties |
defined chemical properties and consistent production |
precise design of mechanical properties and geometric form |
low immune response |
integration of bioactive compounds in the matrix |
Disadvantages of synthetic polymers:
not physiological, does not interact as favorably as biologically derived scaffolds |
only a mechanical scaffold that hold and guide cells in the three-dimensional space until they produce their own matrix environment. |
Biodegradation may induce inflammatory responses |
Limitations of this method:
The current materials used have a lower or lack of contractile function compared to native cardiac muscle, poor tissue morphology, and size limitations. Cardiac myocytes are ischemia-sensitive and need a matrix with high diffusive capabilities for nutrients and oxygen. Myocardium also requires high flexibility, extensibility and high mechanical stability and endurance.
Reasons for these limitations:
engineered matrices resemble diffusion barriers and limit nutrition and oxygen supply in thick constructs (>100-200 um)
current scaffolds do not support the organization of cardiac cells in three-dimensional cardiac tissue constructs in vitro
limited to the mechanical properties of currently used materials
contractile function and actively developed forces of tissue constructs are modest or not detectable
Research achievement with this strategy:
Kofidis T, et al. In vitro engineering of heart muscle: Artificial myocardial tissue. The Journal of Thoracic and Cardiovascular Surgery 2002; 124(1):63-69.
Artificial Myocardial Tissue (AMT) constructs were engineered by seeding neonatal rat cardiomyocytes on a three-dimensional collagen matrix. AMTs were maximally 2.5 mm in thickness and they are grown in dynamic bioreactor with pulsatile flow. Cellular distribution and viability within the artificial myocardial tissues were examined microscopically. The figure below shows seeded cardiomyocytes evenly across the collagen scaffold.
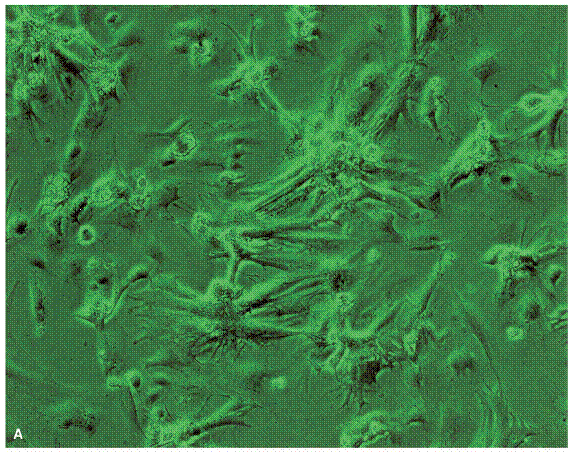
AMTs were cut into strips and examined for contractility. AMTs have a high degree of elasticity. Thy can stretch up to 150% of the original length. In culture, 40% of AMTs displayed continuous contractility at an average frequency of 125 +/- 35 beats/min for about 12 weeks. Force development was analyzed in AMTs, after stretching and after pharmacologic stimulation. After stretching and after pharmacologic stimulation, AMTs displayed enhanced force development. Maximal force of contraction was at 0.02 mN in AMTs. The figure below shows how the force of contraction was measured.
The figure below shows that stretching of AMTs results in increased force development depends on the length of the stretch.
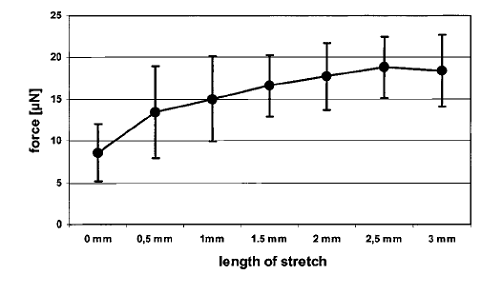
The figure below shows that after stretching and after pharmacologic stimulation, AMTs displayed enhanced force development. The left column is baseline and the right column is stimulation.
Electrocardiograms recorded the spontaneously beating of AMTs of seemly physiological conditions, shown the figure below.
AMTs show promising results for potential use as tissue engineered cardiac muscle replacements. AMTs can be designed into any shape and size to fit the diseased heart. AMTs resemble native cardiac muscle in many aspects such as spontaneous and rhythmic contractions. However, future research needs to be done in stem cell technology and in vascularization of in vitro engineered tissues.
|