DIFFERENT APPROACHES FOR NEURONAL REGENERATION
a. Electric Field
Assisted Neuronal regeneration Approach:
Effect of DC
field (McCaig et.al.)
Ø
Application
of electric fields to damaged neurons represents a new frontier in the
treatment of nervous system injury.
Ø
Several
attempts to enhance mammalian neuronal regeneration with electromagnetic fields
induced by Helmoltz coils as well as with AC or DC fields.
Ø The
first indication that peripheral nerves responded to exogenous electric fields
was discovered during attempts to stimulate regeneration of amputated frog limbs.
When the cathode was positioned at the plane of amputation, as much as 20% of
the distal area of the stump was composed of nerve.
Ø
The
response was dependent on current polarity; in anode-distal stumps and those to
which no current was applied, only 1% of the volume of the limb terminus
consisted of nerve trunks. This result is consistent with the polarity of the
field responses of frog neurones in vitro.
How do electric fields exert their effects in-vivo?
The mechanism
by which electric fields enhance neuronal regeneration remains unclear but
several possibilities exist.
(1) A distally
negative- imposed field reduces inward flow of Ca 2+ at the cut end
of neurons à reduces the level of retrograde
degeneration following transection.
Ø
There
is evidence that an applied field (cathode distal) virtually eliminates Ca2+
influx in lamprey spinal axons and that applied fields augment regeneration of
these same axons (Borgens et al.). Whether these effects are reproduced within
mammalian systems is not known.
(2) The field
may induce branch formation, as has been shown for intact rat peripheral neurons
and cultured frog spinal neurons.
Ø
Increased
branch formation in damaged cells would allow numerous paths to be sampled,
thereby increasing the chances of finding a favorable route for regeneration.
Ø
Alternatively,
uninjured neighboring cells may be stimulated to branch, causing formation of
deviant, yet potentially functional synapses.
(3)
Non-neuronal elements, such as glial cells, fibroblasts or Schwann cells may
respond to the fields, thus affecting the physical composition of the scar at
the lesion site.
Ø
For
example, fibroblasts, which migrate towards the cathode of an applied field,
may be arranged more diffusely. The resulting scar would be less dense,
possibly permitting penetration by advancing growth cones.
Ø
Alternatively,
exogenous fields, which increase capillary permeability, may affect the
inflammatory response following injury.
(4) More
neuroblasts differentiate in the presence of an applied field in vitro so the
field may act as a trophic factor, increasing cell survival and
differentiation.
Example
of in-vivo effects:
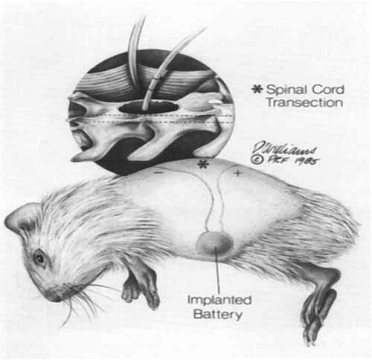
Drawing of
the intraperitoneal battery implantation and subcutaneous electrode routing.
The inset detail shows the approximation of the end of the wick electrode to
the exposed spinal cord at the lamineetomy site. The electrode is sutured to the
axial musculature.
Ø
Using
an implanted battery and electrodes, a weak, steady electrical field across
partially severed guinea pig spinal cords was imposed. Regeneration of dorsal
column axons was observed in experimental animals and sham-treated controls at
50-60 days post-injury by anterograde filling of these axons with the
intracellular marker horseradish peroxidase and by employing a marking device
to identify precisely the original plane of transaction.
Ø In response to electric field
applications, axons grew into the glial scar, as far as the plane of
transaction in most experimental animals. In a few animals, axons could be
traced around the margins of the lesion (but never through it). Moreover, these
fibers returned to their approximate positions within the rostral spinal cord
before turning toward the brain.
Ø In sham-treated controls, ascending axons
were found to terminate caudal to the glial scar, and rarely were any fibers
found within the scar itself. Axons were never observed to cross into the
rostral cord segment. Thus an imposed electrical field promotes growth of axons
within the partially severed mammalian spinal cord, that a steady voltage
gradient may be an environmental component necessary for axonal development and
regeneration, and that some component(s) of the scar impede or deflect axonal
growth and projection.
Ø
Peripheral
nerves within the limb stumps of adult frogs showed an exaggerated growth in
the presence of an imposed field (using implanted DC stimulators). The
regenerative response of lamprey reticu-lospinal neurons was enhanced in-vivo by placing a distally
negative field across the transected spinal cord.
How do electric fields exert their effects in-vitro?
Ø
Applied extracellular steady electric fields of
0.1-10 V/cm have been found (Patel
et. al) to have marked effects on the neurite growth of single dissociated
neurons (Xenopus) in culture.
Ø
Neurites
facing the cathode showed accelerated growth, while the growth of those facing
the anode was reduced.
Ø
Neurites
growing perpendicular to field axis were prompted to curve towards cathode.
Ø
More neurites
appeared to be initiated from the cathodal side of the cell.
Ø
Number of
neurite-bearing neurons per culture & average neurite length increased.
Ø
These effects
are absent in cultures treated with electric fields of similar strength but
alternating polarity and cannot be attributed either to a gradient of
extracellular diffusible substances or to the flow of culture medium produced
by the field.
Ø
Field effects
are reversible: removal of electric field result in loss of neurite orientation
in a few hours; reversal of polarity of the field led to rapid reversal in
neurite orientation.
Ø
Incubation
with concanavalin A (Con A) was found to abolish field effects completely.
Ø Since binding of Con A to neuronal surface has
been shown to prevent field-induced accumulation of Con A receptors toward
cathodal side of these neurons, it is believed that cathodal accumulation of
growth-controlling surface glycoproteins by field is the underlying mechanism
of field-induced orientation of neurite growth toward the cathode.
Asymmetric neurite growth
in vitro in an electric field (5V/cm)
Ø A: bipolar
neuron (1, 2) at onset of experiment. B: after 2 hr exposure to electric field.
Ø Neurite 1 (facing the cathode) grew
substantially, while neurite 2 retracted.
Ø C:
after 4 hr exposure to field. Neurite 1 grew further & branched, and
neurite 2 disappeared. Polarity of field
was reversed immediately after photograph shown in C.
Ø D: 2
hr after the field was reversed, the branched neurite 1 bent toward new cathode
and neurite 2 reappeared on the new cathodal side of the cell.
Ø Note: substantial migration of cell bodies
was observed. Direction of cell migration was towards cathode & cell bodies
appeared to be “dragged” along by growing neurites.
Neural injury
regeneration:
During development, neurons extend long
axonal processes to synapse on specific target neurons. This growth capacity is
lost in the mature central nervous system of mammals so that when an axon such
as an optic axon is severed, it can no longer regrow to restore functional
connections.
However, culture conditions that allow
adult mouse optic axons to regenerate in vitro have been found,
indicating that they retain the inherent capacity to grow. Molecular comparison
with embryonic optic fibers reveal that adult fibers differ in intracellular
axonal proteins and cell surface receptors that regulate growth and mediate interactions
with the glial cells of the mature nervous system. A specific axon-glia /
interactions may be the cause of regenerative failure in adult mammals.
So what is the bottom line?
Ø
It
is hard to dispute the evidence: nervous system tissues, both in vivo and in vitro, strikingly respond to an applied electric field.
Ø
Although
it is not generally appreciated, field effects on cultured nervous tissue can
equal or exceed effects of nerve growth factor (NGF). Cultured dorsal root
ganglia (DRG) demonstrate an enhanced proliferation of processes toward the
negative pole of an applied electric field.
Ø
Moreover,
the very presence of the field has been seen to stimulate neuroblast
development in culture.
Ø
Other
responses of neural tissue to fields include increases in the rate of growth,
increases in the amount of branching of axons, and a decrease in axonal
dieback.
Light assisted neuronal
regeneration Approach:
Ø
Ehrlicher et
al. & Mohanty et al. used
weak optical force produced in optical tweezers by focused laser beam, to guide
direction taken by leading edge of growth cone of cell.
Ø
In actively extending growth cones, a laser spot
placed in front of a specific area of nerve’s leading edge, enhances growth
into beam focus and resulting in guided neuronal turns as well as enhanced
growth.
Ø Power of laser
is so that the resulting gradient forces are sufficiently powerful to bias
actin polymerization driven lamellipodia extension, but too weak to hold, move
growth cone.
Ø Laser Scissors and Laser Tweezers can proved
very useful in studies on neural regeneration. As shown below in the Figure, a
similar analogy can be applied to a neuron where, laser scissor perform the
cutting of the axon, dendrites, etc and laser tweezers can be used to enhance the
regeneration process of the axons and dendrites.
(cellular biophotonics lab, UCI, UCSD)
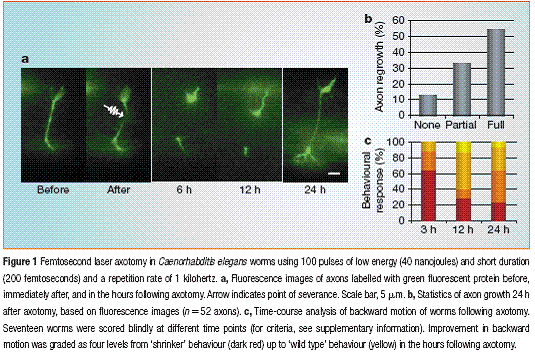
(Yanik et.al Nature 2004)
hybrid approaches
1. Opto-chemotaxis
Light induces chemical modification or releases or activates
caged compounds which act as cues for neuronal growth.
Laser Activated guiding in
agarose hydrogel:
2.. Electro-chemotaxis
=
3. Chemotaxis on patterned
substrates:
Nerves have been directed by topographically structured
artificial surfaces (silicon
wafers or nanotubes) and or by selectively patterning the
substrate with materials that act as adhesives/ attractants for nerves.
Comparison of all approaches:
Chemotaxis approach has high throughput, but
addition and removal of chemical in localized spatial locations with high
temporal resolution is very difficult to achieve.
- It
requires fabrication of special microfluidic channels, valves and pumps.
Guiding neurons
with electrodes in a controlled way also requires pre-fabrication of
microelectrodes on which neurons have to be grown.
- Specific impact of induced electrophoresis
effects is not well understood.
Though
phototaxis approach has advantage of being non-contact in nature, it has very
low throughput.
- Using spatial light modulators (SLM), the laser
beam can be split into several hundreds of moveable trapping beams and thus
throughput of neuronal growth cone manipulation can be enhanced.
Stem cell
approach is very promising as it will rule out surgery and will be minimally
invasive but time could be a constraint. Also it is still in the early research
stage.